My brother and I converted a Kawasaki KX motorcycle with a blown engine to full electric. I will cover the motor specification and acceleration dynamics in some detail here, and briefly touch on the rest of the build process. The dynamics are simplistic (some losses are ignored), but are representative of reality to the extent that they are useful for motor sizing and gear ratio optimization. We re-used two 30V LiPo batteries from an electric car (60V series-output). An LMC Brushed DC motor was donated by a great friend. Specifications from the motor were inputs to a MathCAD worksheet, with which I developed various graphs for optimization of the gear ratio. I optimized the gearing ratio to 3.8, to hit a top speed of 54mph, and a 0 to 40mph acceleration time of 4.57 seconds.

Downloads
Here is a google drive link to my MathCAD worksheet. I will also go through the derivations and explain the graphs in this blog post (so read on for more information).
Motor Specification
This is the area I personally spent the most time, while my brother spent more time on the build. There are a few things to consider when spec'ing out a large electric motor, and it mostly boils down to the torque curves. The torque curves are specified by the manufacturer as an operating threshold. In reality, the primary concern is keeping the motor below the max temperature that it can withstand. There is only so much heat motors can take before components start to fail, such as conductors, potting compounds, electrical contacts, brushes, magnets, or the coils themselves.
There are 3 types of losses that generate this heat:
Resistive heating in the coils and brushes
Eddy currents in the armature
Mechanical losses, primarily bearings
Through the design of a motor, the losses are balanced so that the motor heats up evenly throughout. Small or large, high speed or high torque motors will each be designed with different requirements, and balancing these losses. All of this boils down to torque curves for the practical end use.
Here are all the parameter inputs, equations, and torque curves for the motor we selected (well, that was donated) for our motorcycle. It is the LMC200-RAGS:


This torque curve is not exemplary for all motors, but I have learned that it is typical of a large motor. The flat top, that quickly drops off near max rpm, is due to the low coil resistance of the LMC200 (16.95 mOhm). This motor is designed to run in the transient phase (accelerations) with massive current and torque. Low coil resistance reduces resistive losses. It is an air cooled motor, so it is best to get the motor up to speed as quickly as possible, and not so good at low speed, high torque applications.
Peak Current & Torque: This is short term, burst power from the motor. Good for accelerations, and should be rated for ~ 5 seconds (after which the motor could heats up too much).
Continuous Current & Torque: This is the current and torque that the motor should be able to sustain indefinitely, given a reasonable operating environment (good cooling).
Nominal Torque @ RPM: This is the operating point that the motor is designed to work best at. Typically, this is also the maximum efficiency point. The gearing should be spec'd as to keep the motor comfy around here.
No Load Speed: This is good to double check that your calculations are representative, but doesn't mean anything for actual operation.
Stall Current & Torque: This is the torque at which the motor will stop spinning, while supplying it with the rated voltage. You definitely want to avoid doing this during normal operation.
Acceleration Dynamics
A free body diagram (FBD) is shown here to create a torque balance between the motor "tau_m", and the tire, or load "tau_l".


This differential equation is of the form:
There is actually a closed-form solution to this non-linear differential equation. It is:
Using the closed-form solution is OK if the inputs are all continuous, but if you require discontinuities (such as a peak-to-continuous current step), a numerical solver is easier. Here is my MathCAD sheet with a solve block that allows me to have discontinuities that you will notice in the graphs.

At this point, I was able to visualize the acceleration profile using the solution of this solve block. With a few iterations, I quickly optimized the gear ratio " r " for my requirements.
From 0 to 5 seconds, the motor is operating at its peak current limit of 365 amps. After 5 seconds, the current drops to the continuous limit of 200 amps. Then, at around 8 seconds, the battery voltage of 60V is reached. It's clear that the current dictates the acceleration (because motor torque is proportional to current), and the battery voltage dictates the top speed (because motor speed is proportional to voltage).


Sub-Systems
Here are the sub-systems, with a few useful part numbers. The total cost for major components (excluding the batteries and motorcycle frame) was approximately $3000 dollars.
Drivetrain
BDC Motor: LMC200-D135RAGS ($1800)
Front sprocket with custom, tapered bore
Controls
Motor Controller: Alltrax SR ($716)
Throttle: domino, Comando Acceleratore ($129)
Monitor: Grin Technologies, Cycle Analyst V2.3 ($100)
Power Electronics
Power Cables
Main Disconnect: Tyco Electronics, KILOVAC EV200 ($125)
Ammeter Shunt ($45)
LiPo Battery Packs (2x, 60V series-output)
Structures
Kawasaki KX Frame
Custom Battery Mounts
Build Pictures
The motor mounts were quite ad hoc. We chopped up whatever aluminum plates we had laying around and fit them to the LMC motor by eye. I call this "sharpie machining". Original engine mounts were re-used.
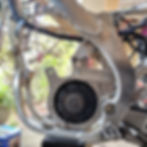
Here is my brother...cutting a check. In the foreground, there is the mess of wires that are being organized for the control (key, throttle, and switches).
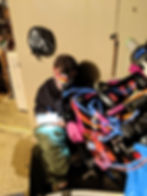
This Alltrax SR controller was quite easy to program and wire up. It allows for some basic tuning, such as custom throttle curves, continuous current, peak current (and time), etc.

Below, you can see just how naked a motorcycle looks without all the internal combustion bits. No gas tank, no engine, no radiator, no carburetor/injector, no air filter, etc.

Each battery fits inside of a pelican case, and the case is mounted to the back of the bike. I was actually against this, because the pelican cases add significant mass to an already heavy battery pack. My brother won this disagreement. The advantage is that the batteries are fully waterproof, heavily protected, and contained (in the case of a battery fire). Reinforcements were added to the rear structure to carry the extra weight.
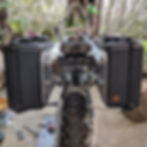
Below, you see the bike near completion. You can also see the backside of the LMC motor, and note that it is air cooled. In my opinion, an air cooled motor is not ideal for a utility or trail riding motorcycle (such as this one). Riding the bike around our property, I noticed it was overheating when moving at slow speeds under load (full bag of tools, or a passenger). Eventually, I burned it up!
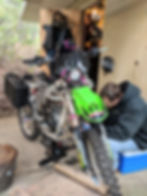
These are the brushes for the LMC. When I was riding up a hill, loaded up with a passenger, and slowly through obstacles, the motor overheated. The failure point, or weak link, were the brushes. You can see that one of the aluminum brush holders actually melted. Also, the brushes look heavily oxidized, which would have increased the electrical resistance, and caused further heating (run-away heating).
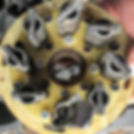
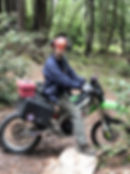
Thank you for reading!